THIS ARTICLE/PRESS RELEASE IS PAID FOR AND PRESENTED BY SINTEF - read more
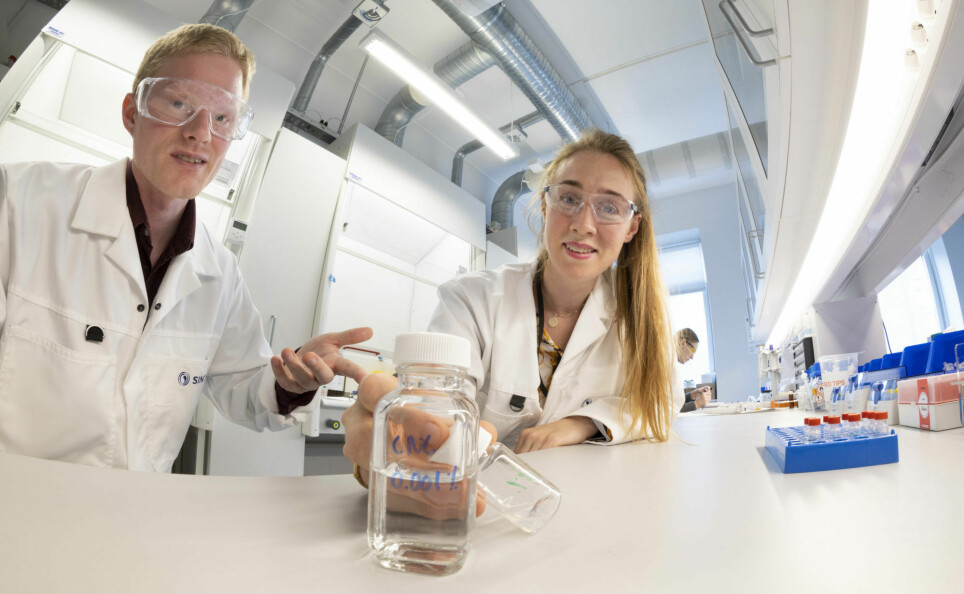
Shedding light on Nature’s tiniest building blocks
These research scientists are studying Nature’s own nanomaterials – applying tools and methods that are normally used for something quite different. Their work has provided us with knowledge that may revolutionise everything from medical treatments to building constructions.
“First we have to talk about what we mean by nano,” says SINTEF researcher Sigurd Wenner. “Many people regard nanotechnology and nanomedicine as sinister or dangerous, but this simply isn’t true. Nano is simply a prefix for measurement units denoting something very, very small. For example, if you have walked one thousand metres, you can also say that you have covered one kilometre. This isn’t far, but for practical reasons, we use ‘kilometre’ to denote the distance. We use the prefix ‘nano’ in the same way as we use ‘kilo’.”
Sigurd and his fellow researchers at SINTEF are developing entirely new methods to help us understand the structure of organic nanomaterials, and nanotechnology will provide us with new knowledge that will enable us to find applications for a variety of these materials – particularly in the field of medicine. The project has been given the name MOSON (Molecular Structure of Organic Nanomaterials).
“Some tools are like mechanical diggers. They work well for excavating rock and gravel but are entirely unsuited for uncovering a Viking longship.”
No Covid vaccine without nanomaterials
Nano is from the Greek ‘nanos’, meaning dwarf. A nanometre is one billionth of a metre. Nanotechnology thus involves objects that are extremely small, and which require highly advanced investigative and analytical techniques.
Nanomaterials can be extremely useful in medical applications. If you have had your Covid jabs, you have probably already come into close contact with this technology. Modern mRNA vaccines carry the recipe mRNA molecule into your body attached to a part of the virus’ protein. Your body then manufactures the protein that enables your immune system to respond by producing antibodies to combat the virus. In this way we train our bodies to recognise the Covid-19 virus, effectively equipping it to fight off an attack if you become infected.
“But the delivery of mRNA would not be possible without nanotechnology,” explains Birgitte McDonagh, Wenner’s colleague at SINTEF. “mRNA is a very delicate and poorly resilient molecule that must be carefully protected and packaged in order to reach its target in our bodies. In order to protect the mRNA in the vaccine, it is encapsulated in minute fatty globules called lipid nanoparticles, which act as the carrier media for the mRNA molecules. The nanoparticles ensure that the mRNA is protected and can be taken up by our bodies’ cells,” she says.
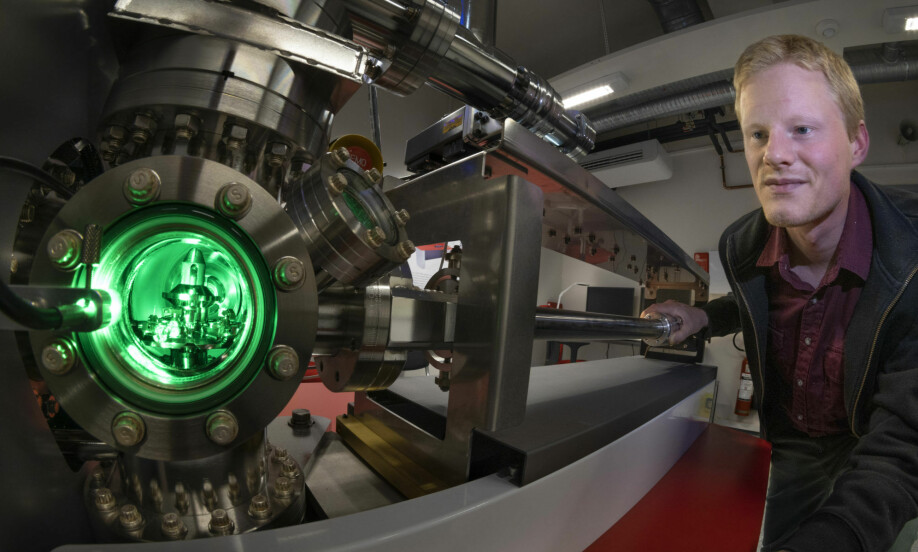
From trees to bone reconstruction
There are also other organic nanomaterials that have useful applications in medicine. One of these is nanocellulose, which is the building block of wood fibre.
“Cellulose is one of nature’s strongest materials and is the reason why trees can grow to be tens of metres tall and remain standing in the face of very high winds,” says McDonagh. “While nature has had millions of years in which to develop these materials, we scientists can save time by applying them directly,” she says.
“Part of the key to the properties of any material is its crystalline structure. For example, we can compare graphite and diamond, which are both made of the same element – carbon. However, they have different crystalline structures. Graphite is soft and dull and is used to write on paper, while diamond is very hard and brilliant, and valued as a precious stone.”
If we separate wood fibres into their smallest constituents, we are left with microscopic fibre-like splinters called nanocellulose, which are ten thousand times thinner than a human hair. Even though these splinters are very small, they are extremely strong.This strength, among other things, makes them interesting for use in medical research.
In the lab, scientists can shape nanocellulose fibres into dry and porous composite materials with multiple applications.
“Nanocellulose is a fantastic material,” says McDonagh. “Not only is it strong, but there is an almost unlimited supply and, perhaps most important of all, it is seen as safe in contact with humans. For this reason, a lot of research is being carried out into the use of nanocellulose networks as a form of ‘scaffolding’ on which to base bone reconstruction,” she says.
The idea is that nanocellulose can be introduced into a bone fracture in order to assist the bone cells to grow and repair the break. In the international research community this process is known as tissue regeneration, the aim of which is to help the body to repair injury and damage.
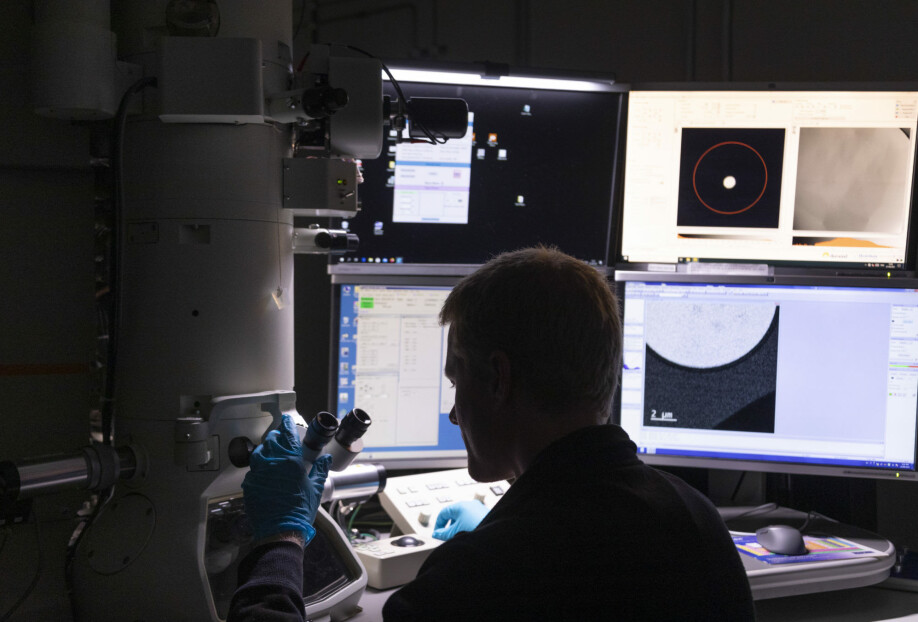
Basic research into organic nanomaterials
These are just two examples of organic nanomaterials that we know possess unique properties. There are many such materials, although we know relatively little about most of them. This is where the Norwegian scientists and their strategic project MOSON come in.
“As part of this project, we’re planning to analyse the properties of a number of different organic nanomaterials,” says Project Manager Sigurd Wenner. “The materials’ chemistry and atomic structure will determine their properties and what they can be used for,” he says.
What we are talking about here is basic research, where the aim is to offer new insights into the properties of materials that may have multiple future applications, but which have no immediate impact on existing technologies.
The project has now reached the half-way stage and the researchers have already obtained some good results in their search for a new window onto their nanoworld.
Nanoworld – where a grain of sand seems very big
A full understanding of these minute building blocks requires special methods and, not least, equipment that makes it possible to see how the materials are structured, as well as determine their chemical properties. This all happens at a scale that is beyond our imaginations. We are back in the nanoworld.
The particles we are talking about are no bigger than about 100 nanometres, meaning that the smallest grain of sand that you can see with the naked eye will occupy the space taken up by one billion of these particles.
The tools employed by the MOSON project scientists are instruments normally used to study the structures and chemical compositions of hard and resilient materials such as steel, rock and electrical components. Such materials are easy to handle and to cut into smaller pieces for investigation in a multitude of ways. But the project team is now focusing on softer materials.
“Some tools are like mechanical diggers. They work well for excavating rock and gravel but are entirely unsuited to uncovering a Viking longship,” says Wenner. “That’s why we’re developing entirely new and gentle ways of using the equipment that are better adapted to investigating these delicate organic nanomaterials. Instead of bombarding the materials with a jackhammer, we’ll be using the instrument in the same way as microbrushes are applied in an archaeological dig,” he says.
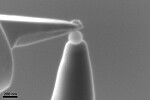
‘Bulletproof’ capsules
One of the project’s key prerequisites is to ensure that the organic nanomaterials can withstand electron bombardment and high vacuum conditions. For this reason the researchers have applied a number of encapsulation techniques in order to protect the materials.
One of these involves encapsulation in so-called ‘water glass’, which is a form of glass derived from an aqueous solution. This technique enables analysis of the structure of biomolecules such as proteins, which are intolerant to dehydration.
“As a model material for this encapsulation technique we have used proteins attached to a particle of gold. We have succeeded in demonstrating that we can protect the proteins during electron imaging,” says Wenner “The gold particles act as a target indicating where the proteins are located, while the water glass acts as their ‘bulletproof vest’,” he explains.
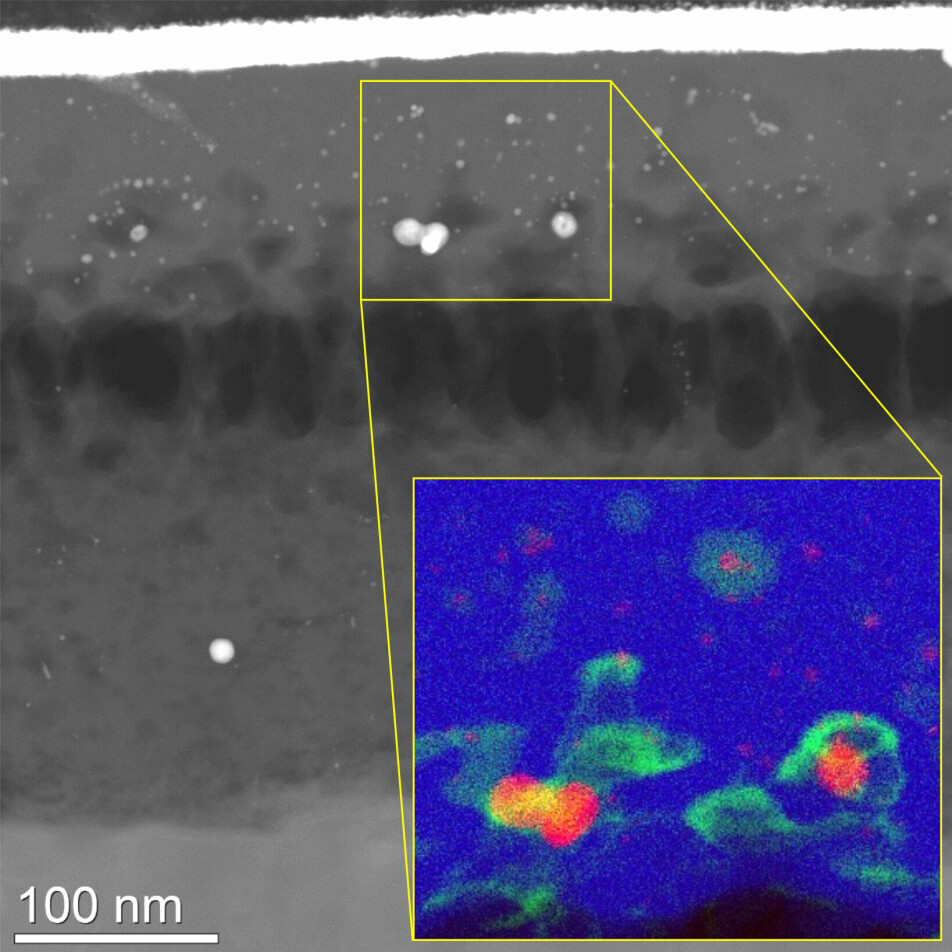
Crystalline structure is the key to strength
“A material’s crystalline structure can be key to its physical properties,” explains Birgitte McDonagh. “For example, we can compare graphite and diamond, which are both made of the same element – carbon. However, they have different crystalline structures. Graphite is soft and dull and is used to write on paper, while diamond is very hard and brilliant, and valued as a precious stone,” she says.
The crystalline structure of a material can be determined using electron diffraction, which is used traditionally to investigate the structure of metals, semiconductors and minerals. The method can also reveal material properties such as strength or electrical conductivity.
But what is a crystal? A material’s crystalline structure reflects how its atoms are organised. Glass and gypsum have no crystal form because their atoms are completely disorganised. In a true crystal atoms are arranged in organised three-dimensional lattices. These lattices of atoms form repeating patterns such as we see in chicken wire or knitwork. Crystals are not necessarily all shiny and beautiful. What we call ‘crystal glass’ actually has no crystalline structure, whereas any ordinary rock or tin can does. Even cellulose has a crystalline structure!
Wenner continues: “During electron diffraction, the atomic lattice in a crystalline structure acts as a collection of mirrors that reflect the electrons as they pass through it. We can determine the crystal structure of a material by analysing the directions in which a beam of electrons are scattered. This in turn provides us with more information about the material’s properties.”
Examining nanoparticles one by one
Perhaps an even more amazing thing about electron diffraction is that an electron beam can be focused to target a single nanoparticle at a time.
This approach is called nano-electron diffraction and is used to determine the extent to which a particle is crystalline. In practice, this can tell us how robust a nanocellulose structure is, and from this we can determine its resilience and what it can be used for. There are in fact many different types of nanocellulose, with many different applications.
However, our constant challenge is the delicate nature of organic materials. We are only able to send a few thousand electrons through a particle before its structure is destroyed.
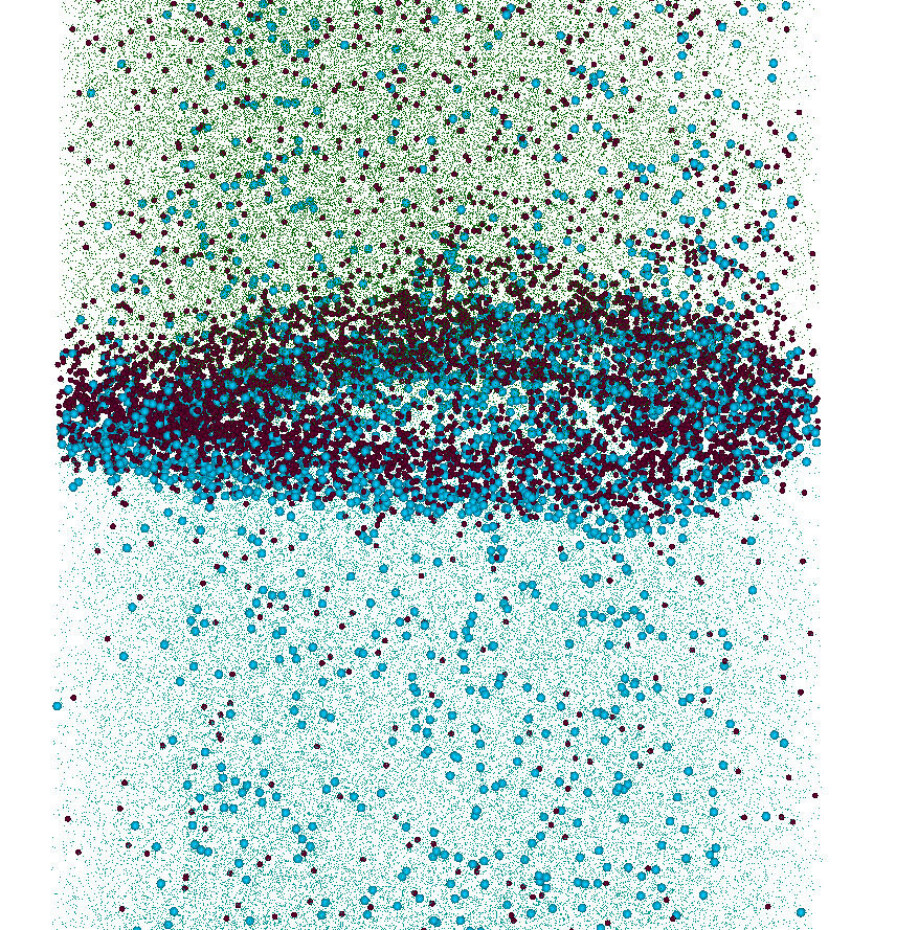
“This is why it is important to capture every single electron that has passed through the particle, and obtain as much information as we can from it,” says Wenner. “By using an electron sensitive camera we can see in which directions the electrons are reflected and this gives us a pattern that tells us what type of crystalline structure the particle has. We’re able to do this by using a new generation of cameras developed originally at CERN for particle physics research, and are currently working to adapt the technique to the nanoscale study of organic materials,” he says.
Constituent elements determine chemical properties
While electron diffraction measures how the atoms are organised in a material’s crystal lattice, a technique called atom probe tomography is used to determine its constituent elements.
“This method enables us to determine a material’s constituent elements and the position of individual atoms in three dimensions,” says Wenner. “It effectively evaporates the atoms from a material, one atom at a time, after which we construct a 3D model of a single nanoparticle and study it in detail,” he says.
This enables researchers to identify what atoms are attached to the surface of particles such as cellulose crystals or lipid nanoparticles, which are used as carriers for the Covid vaccine.
Surface chemistry is important in medical applications in particular, because it is the surface of the nanomaterial that first comes into contact with our bodies’ cells.
“So, what we’re doing in practice is developing our expertise and contributing towards techniques that will be of major significance to the medical sector,” says Wenner. “But they may also be of benefit in other fields, such as the study of nanoplastics. These techniques enable us to examine different types of plastic and to obtain information about how plastics decompose and the extent to which they disperse in different environments,” he says.
———
Read the Norwegian version of this article at forskning.no
See more content from SINTEF:
-
Norwegian boys dislike school the most
-
Old brickwork can be brought back to life in old heritage buildings
-
Researchers surprised by the reactions of penned fish to robots
-
Researchers have run over 1,000 tests on Norwegian windows: This is why rainwater leaks into homes
-
Is it safe to feed farmed salmon with bristle worms raised on fish waste?
-
A solar storm can render your phone useless