THIS ARTICLE/PRESS RELEASE IS PAID FOR AND PRESENTED BY NTNU Norwegian University of Science and Technology - read more
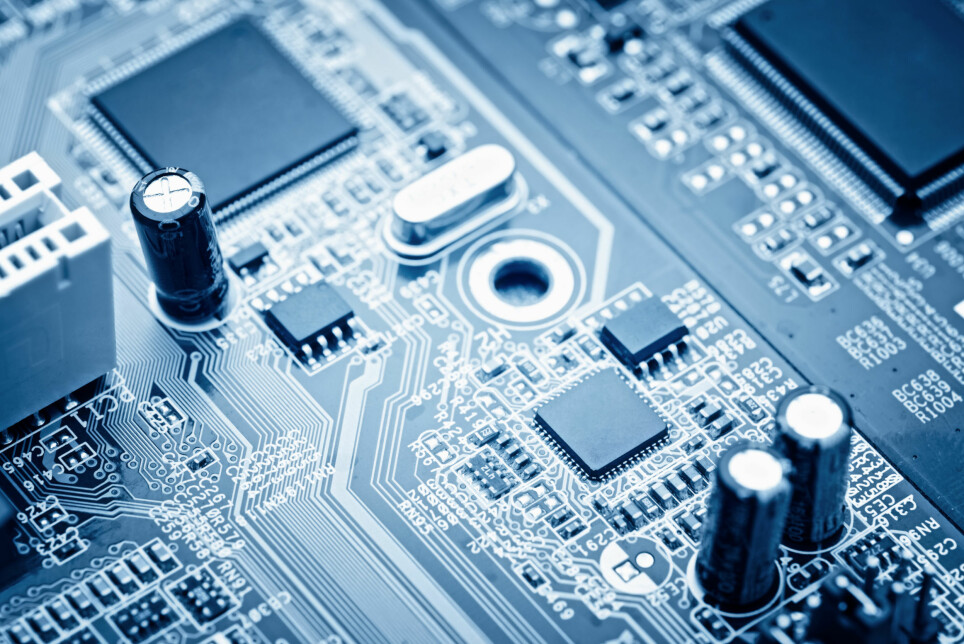
A new way to look at the inner workings of tiny magnets
Movies of micromagnets created by researchers at NTNU could further our understanding of materials for the next generation of computers.
Researchers from NTNU are shedding light on magnetic materials at small scales by creating movies with the help of some extremely bright x-rays.
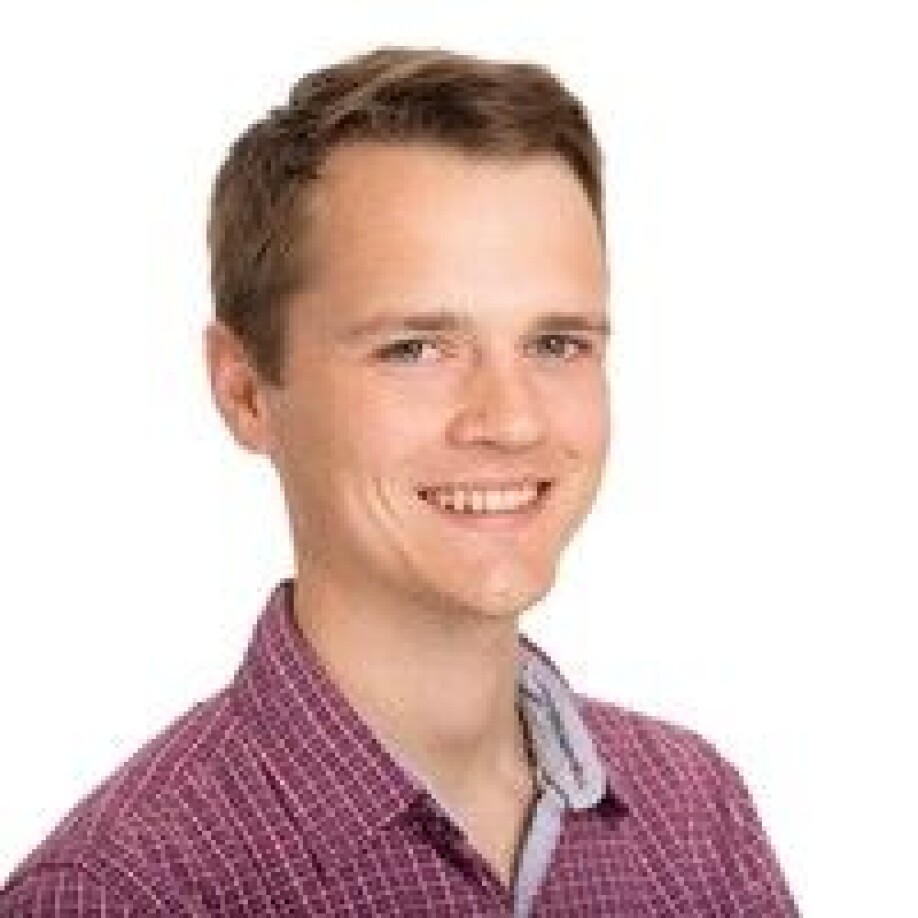
Erik Folven, co-director of the oxide electronics group at NTNU’s Department of Electronic Systems, and colleagues from NTNU and Ghent University in Belgium set out to see how thin-film micromagnets change when disturbed by an outside magnetic field. The work, partially funded by NTNU Nano and the Research Council of Norway, was published in the journal Physical Review Research.
Tiny magnets
The tiny square magnets, created by NTNU PhD candidate Einar Standal Digernes, are just two micrometers wide and split into four triangular domains, each with a different magnetic orientation pointing clockwise or anti-clockwise around the magnets.
In certain magnetic materials, smaller groups of atoms band together into areas called domains, in which all the electrons have the same magnetic orientation.
In the NTNU magnets, these domains meet at a central point – the vortex core – where the magnetic moment points directly in or out of the plane of the material.
“When we apply a magnetic field, more and more of these domains will point in the same direction,” says Folven. “They can grow and they can shrink, and then they can merge into one another.”
Electrons almost at the speed of light
Seeing this happen isn’t easy. The researchers took their micromagnets to an 80m-wide donut-shaped synchrotron, known as BESSY II, in Berlin, where electrons are accelerated until they are travelling at almost the speed of light. Those fast-moving electrons then emit extremely bright x-rays.
“We take these x-rays and use them as the light in our microscope,” says Folven.
Because electrons travel around the synchrotron in bunches separated by two nanoseconds, the x-rays they emit come in precise pulses.
A scanning transmission x-ray microscope, or STXM, takes those x-rays to create a snapshot of the material’s magnetic structure. By stitching these snapshots together, the researchers can essentially create a movie showing how the micromagnet changes over time.
With the help of the STXM, Folven and his colleagues disturbed their micromagnets with a pulse of current that generated a magnetic field, and saw the domains change shape and the vortex core move from the centre.
“You have a very small magnet, and then you poke it and try to image it as it settles again,” he says. Afterwards, they saw the core return to the middle – but along a winding path, not a straight line.
“It will kind of dance back to the centre,” says Folven.
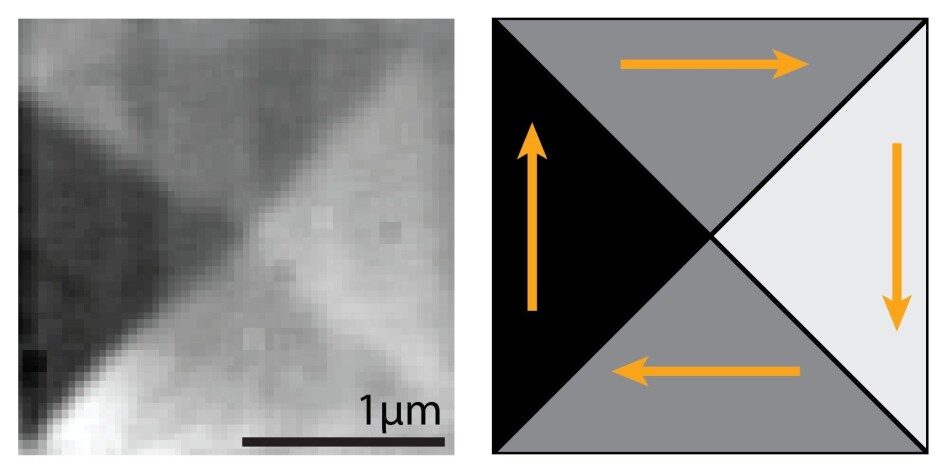
One slip and it’s over
The team’s biggest challenge was making the samples in the first place. “We were very, very uncertain whether it would even be possible when we started out,” says Folven.
That’s because they study epitaxial materials, which are created on top of a substrate that allows researchers to tweak the properties of the material, but would block the x-rays in a STXM.
Working in NTNU NanoLab, the researchers solved the substrate problem by burying their micromagnet under a layer of carbon to protect its magnetic properties.
Then they carefully and precisely chipped away the substrate underneath with a focused beam of gallium ions until only a very thin layer remained. The painstaking process could take eight hours per sample – and one slip up could spell disaster.
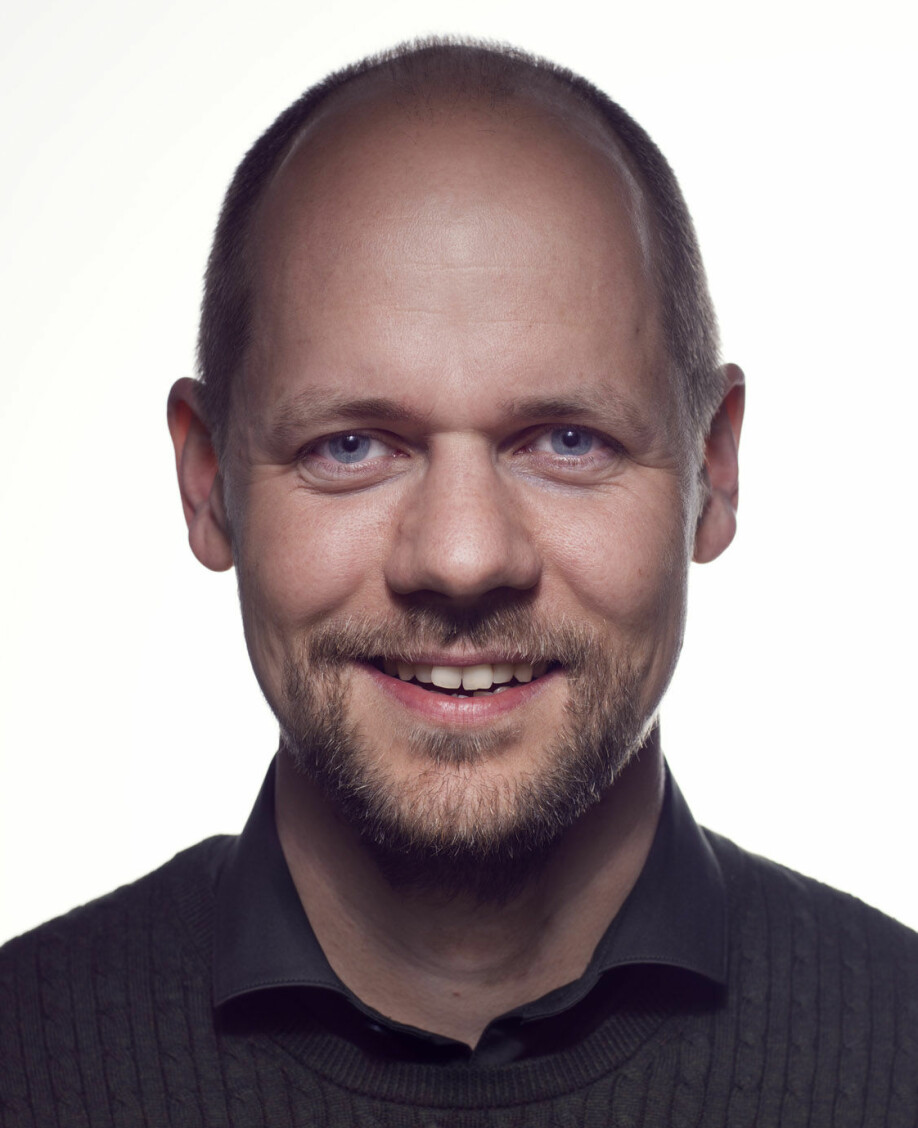
“The critical thing is that, if you kill the magnetism, we won’t know that before we sit in Berlin,” he says. “The trick is, of course, to bring more than one sample.”
From fundamental physics to future devices
Thankfully it worked, and the team used their carefully-prepared samples to chart how the micromagnet’s domains grow and shrink over time. They also created computer simulations to better understand what forces were at work.
As well as advancing our knowledge of fundamental physics, understanding how magnetism works at these length and time scales could be helpful in creating future devices.
Magnetism is already used for data storage, but researchers are currently looking for ways to exploit it further. The magnetic orientations of the vortex core and domains of a micromagnet, for example, could perhaps be used to encode information in the form of 0s and 1s.
The researchers are now aiming to repeat this work with anti-ferromagnetic materials, where the net effect of the individual magnetic moments cancels out. These are promising when it comes to computing – in theory, anti-ferromagnetic materials could be used to make devices that require little energy and remain stable even when power is lost – but a lot trickier to investigate because the signals they produce will be much weaker.
Despite that challenge, Folven is optimistic. “We have covered the first ground by showing we can make samples and look through them with x-rays,” he says. “The next step will be to see whether we can make samples of sufficiently high quality to get enough signal from an anti-ferromagnetic material.”
Reference:
Einar Digernes et.al.: Direct Observation of Temperature Dependent Vortex Dynamics in a Micromagnet. Physical Review Research, 2020.
See more content from NTNU:
-
Surprising findings about ADHD may help girls who are struggling
-
Exercise helps improve how our brain ages
-
Is your washing machine built to have a shorter lifespan than it used to?
-
New clues from old bones: Some Vikings were much more violent than others
-
People with a positive attitude are built differently
-
Board composition: This is crucial for whether companies take social responsibility