An article from University of Oslo
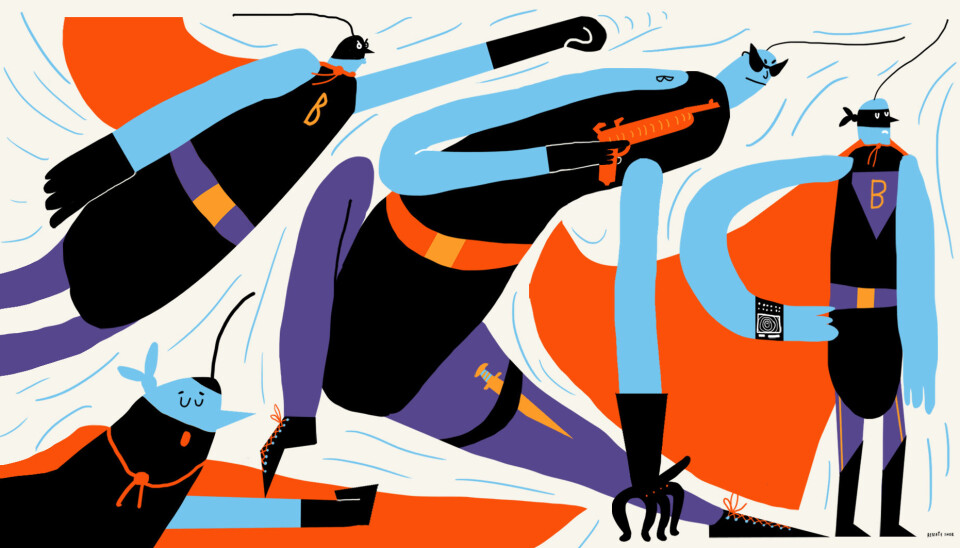
Bacteria: The new superheroes
How ambitious researchers use computers to push bacteria beyond their limits.
Bacteria are everywhere. They colonize our skin and crowd our intestines, easily matching the total number of human cells in the body. Bacteria also live at the bottom of the oceans, survive decades frozen in the ice, and flourish deep, deep underground. They’ve been doing so for billions of years, and they will continue to thrive long after we are gone.
For the most part, bacteria have a poor reputation, and symbolize disease, death and decomposition. They keep multiplying, eating away at skin, wood and metal, and rapidly evolve defenses to medical interventions. At the same time, bacteria and science share a long history of collaboration. For decades, scientists have harnessed bacterial talents. Now, this duo has included a third partner: computers.
Bob the builder
Computational approaches have opened up a whole range of possibilities for biotechnology, where ambitious scientists push bacteria beyond their limits.
"We want to use bacteria to produce concrete," says Anja Røyne at the Department of Physics at the University of Oslo, lead researcher for BioZEment2.0.
She describes a future where bacteria produce construction materials for houses and roads. Røyne has a background in physics, and hopes to tackle a big problem in industrial production of concrete: large carbon emissions from factories that burn limestone to get the reactive raw material needed to glue together sand grains in the concrete.
Can Røyne manage to convince bacteria to do the same job, carbon emission free?
Together with a large team she aims to facilitate a collaborative effort between two populations of bacteria. The first population of microorganisms bathe in a sugar-rich substrate as they deconstruct limestone into calcium and carbonate ions. Another batch of bacteria use the dissolved limestone to build solid bridges between sand grains. The result: a solid block of concrete produced by bacteria.
Digital bacteria
"The first results were not great, and I wouldn’t build a house with the very first concrete from the lab. But our results show that the process can work," comments Røyne.
Funding from Digital Life Norway (DLN) will allow the researchers to understand and shape the living production facility. A first step is to work with digital bacteria to understand the chemistry and physics behind their capabilities.
"We are currently developing two computer models. One is to test how bacteria, nutrients, water, and sand grains behave at a small scale. A second model explores the genetic and metabolic processes inside the bacteria," says Røyne.
Alexander Wentzel is a key collaborator in the project, and works out of his laboratory at SINTEF in Trondheim. The region has also contributed with local bacteria to the project.
"We needed bacteria that would be able to live in high pH environment, so I went on an expedition close to a limestone quarry to collect samples. In the soil samples we found bacteria that can take part in the bio-concrete production," explains Wentzel.
An ocean of possibilities
A second, and much larger, bacterial harvest comes from the fjord outside Trondheim. SINTEF’s current collection contains many thousand marine bacterial strains. 1200 of the bacteria have had their genome sequences determined.
Wentzel and his team will use the data from the marine bacteria to discover novel antibiotics, a DLN-project called INBioPharm. By mapping candidate gene clusters with digital tools, and then testing the potential genes in host bacteria in the lab, Wentzel hopes to make discoveries that are relevant for the pharmaceutical industry.
"We also develop bioinformatics tools that will be valuable for other projects," he comments.
Combining computational power and experimental work to discover interesting genes and biomolecules is relevant for research projects within health, industry, and agriculture.
Woodwork
Biochemist Vincent Eijsink at NMBU in Ås is not primarily interested in antibiotics, but rather in bacterial enzymes.
"We aim to combine digital models with laboratory work to understand how specialized bacterial enzymes work together to degrade plant material," says Eijsink.
In his project, the aim is to discover and develop enzymes that can turn otherwise wasted biomass into useful products. Both of these projects rely on data from the marine bacteria collected by Wentzel and his team, but the detailed algorithms and secondary databases that help identify candidate genes or enzymes are different.
The project at NMBU has recently started, and Eijsink estimates that it will take a few years to get the models up and running. Then, he and his colleagues will be able to start modifying the digital and living bacteria, and optimize enzyme systems needed for biomass processing.
"We aim to recycle or refine plant material from forestry or food production into usable products. Enzymes can do the job for us, and the bacteria teach us how to optimize our processes," says Eijsink.
Golden microorganisms
Another job easily performed by bacteria, is extracting metals from their surroundings.
"Bacteria will extract palladium or gold from their environment and reduce the metals to nanoparticles," explains Dirk Linke at the Department of Biosciences at UiO.
He and his co-workers screen the properties of bacteria with random mutations, aiming to develop nanoparticles for the oil industry.
The tiny particles produced by bacteria often show interesting catalytic capabilities. The goal of Linke’s project is to modify and fine-tune the bacteria so that they produce novel and potentially useful nanoparticles.
"There may be new and interesting properties to nanoparticles when you can fine-tune size and composition, and combine different metals to get nanoparticles with specific purposes," he says.
Bridging the gap
In the lab Linke and his team test how different populations of bacteria can absorb, reduce and deposit the metals as nanoparticles. Resulting experimental data provides the basis for the computer models.
Linke, Røyne and Wentzel all collaborate with systems biologist Eivind Almaas at Department of Biotechnology and Food Science at NTNU, an expert in bridging the gap between experimental and digital research.
"Systems biology integrates experimental data and computer models to extract more and better data from the research projects," says Almaas.
The interdisciplinary teams collaborate closely, and use output from digital models to prioritize what experiment to do next. This approach saves the researchers time and energy, and may also point to avenues of research where big discoveries are more likely.
Embedded in each research project is the open question of how bacteria will affect society in the future. Other challenges include the size of the computer models and data sets, how to provide enough food for bacteria in industrial-scale setups, and how to navigate the biotechnological regulations of genetically modified bacteria.
The digital life science community will need more than computers to meet these challenges.