THIS CONTENT IS BROUGHT TO YOU BY University of Oslo - read more
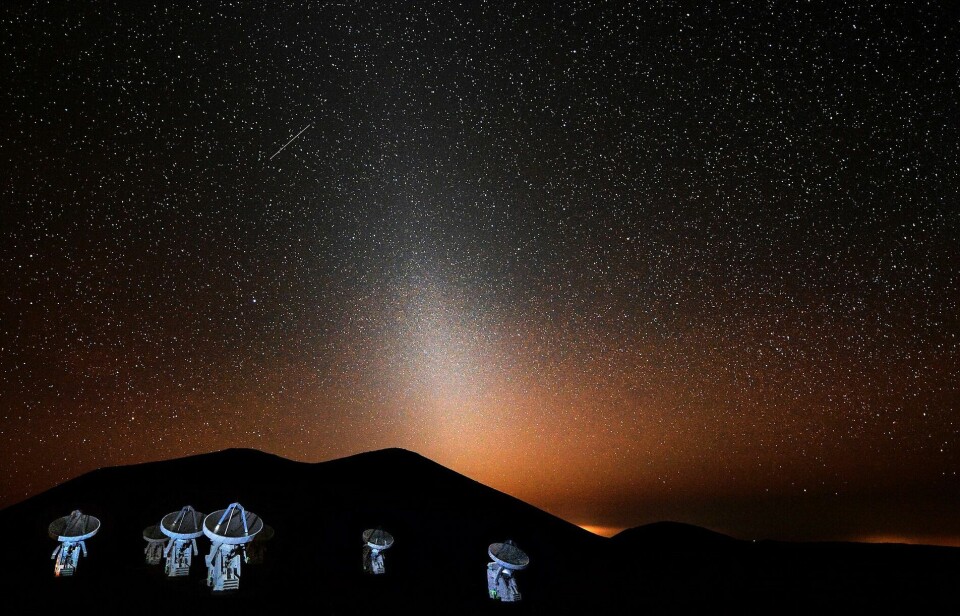
A cloud of dust prevents us from seeing the universe clearly – researchers are now going to clear it up
The researchers will map the dust so we can get a clearer picture of the universe.
Cosmic infrared radiation gives us a picture of everything that has happened in the universe from a few hundred thousand years after the Big Bang until now. However, dust in our own solar system emits light at the exact same wavelengths, disrupting the image.
Researchers will now use historical data from various telescopes to get a clear picture of what is dust and what is actually radiation from space beyond our galaxy.
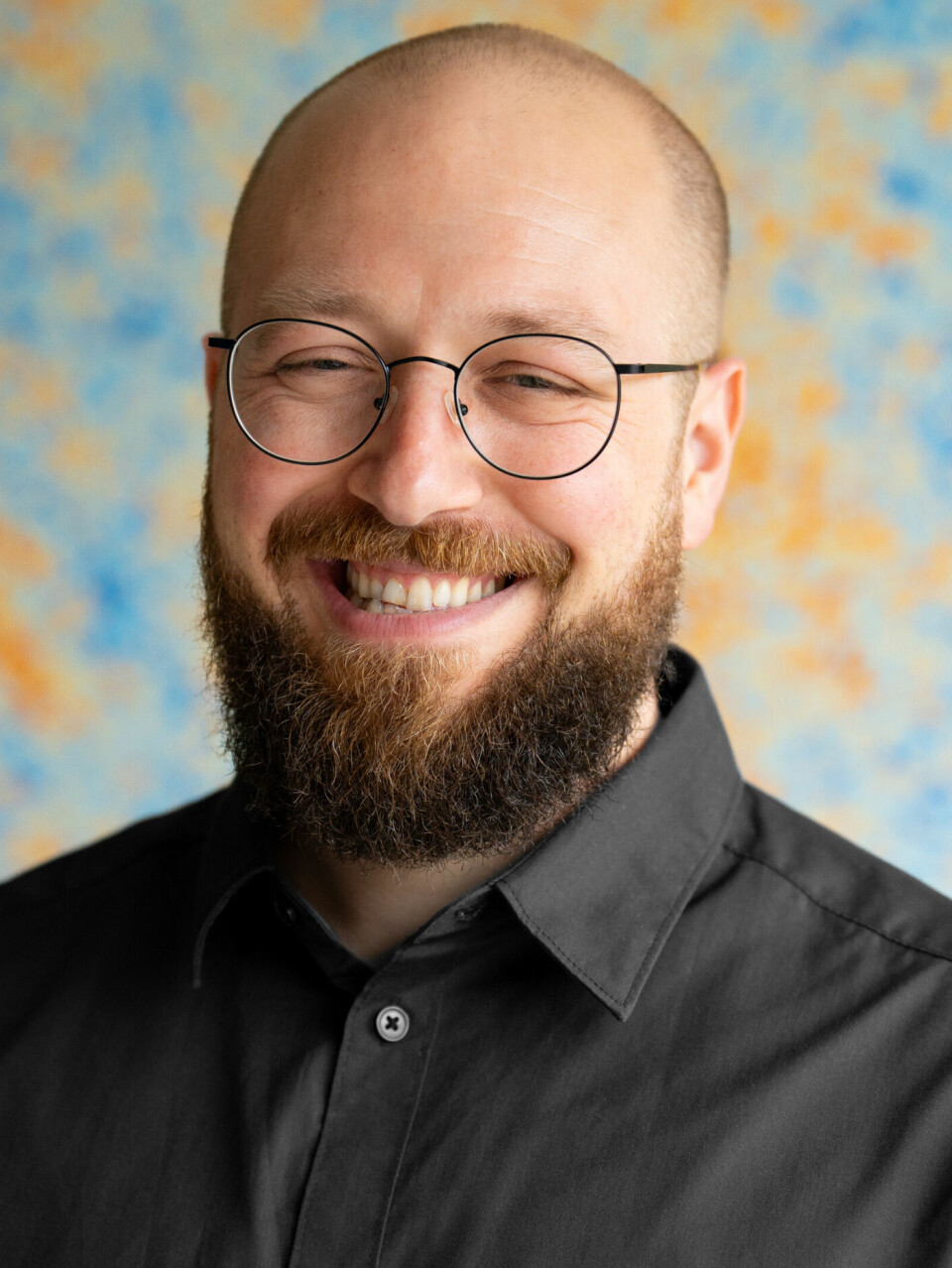
“Our main goal is to create maps of all infrared galaxies in the entire universe, from the very first galaxies up until today,” says Duncan Watts.
He is a researcher at the University of Oslo's Institute of Theoretical Astrophysics.
With maps showing the cosmic infrared background radiation, researchers will be able to determine when and where galaxies formed. They will also be able to understand the physical processes behind them.
This research will also contribute to knowledge about our own local galaxy, the Milky Way, and our own solar system.
“To create these maps, we will map the interplanetary dust cloud that surrounds our solar system. This involves understanding how asteroids, comets, and other small objects contribute to this emission,” says Watts.
Radiation from the dawn of time
Infrared radiation, or infrared light, is electromagnetic radiation with wavelengths between 0.7 micrometres and 1 millimetre.
While the stars we see in the sky are hot enough to emit visible, high-frequency white light, objects with lower temperatures emit infrared, invisible light.
Today, 13.7 billion years after the universe’s birth and the Big Bang, space is filled with cooled dust that emits this infrared light. This dust originates from the formation of stars and galaxies from the dawn of time until today.
Watts compares it to the faint glow from the embers of a campfire. Cosmologists call it CIB – cosmic infrared background light.
“If we look at the big picture, we can still see the light from the Big Bang. Right after the Big Bang, all the mass was so densely packed that light couldn’t escape. After about 300,000 years, the universe had expanded enough for light to break free,” explains Watts.
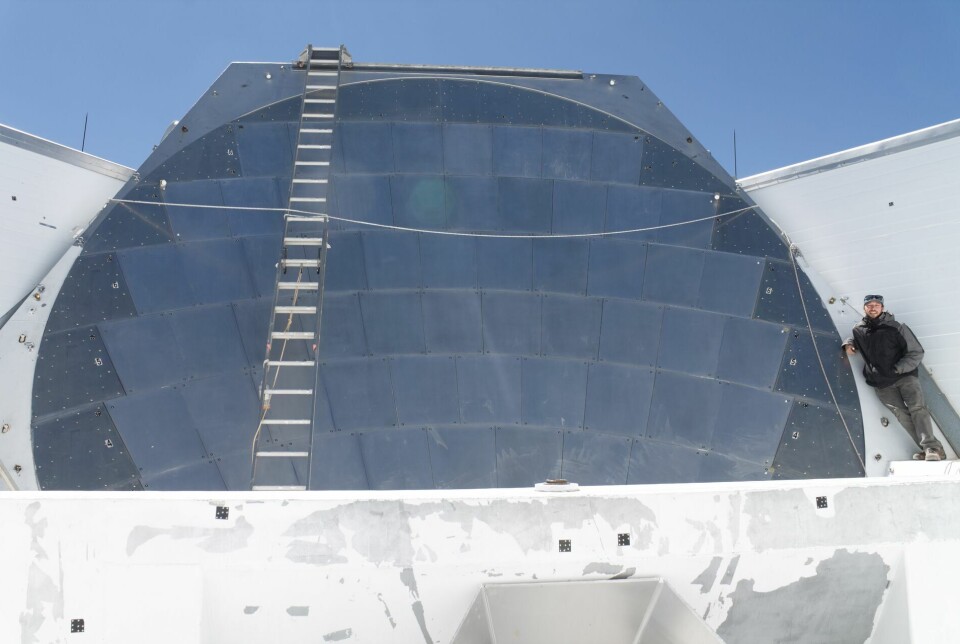
Since then, this light has continued to spread through space. Over time, thanks to the universe’s constant expansion, the light has been stretched into longer and longer wavelengths – it has become redder.
The very first light waves released after the Big Bang have now actually turned into microwaves, with wavelengths of several centimetres.
The weak background radiation
The infrared light that Watts is interested in originates from events that occurred some time after the Big Bang, from when the first stars were formed and the first galaxies took shape.
“Most research on early galaxies has focused on looking at one galaxy at a time. But if you use only one telescope, like the Hubble Telescope, there will often be so many galaxies in the field of view that they overlap and can’t be distinguished from each other,” says Watts.
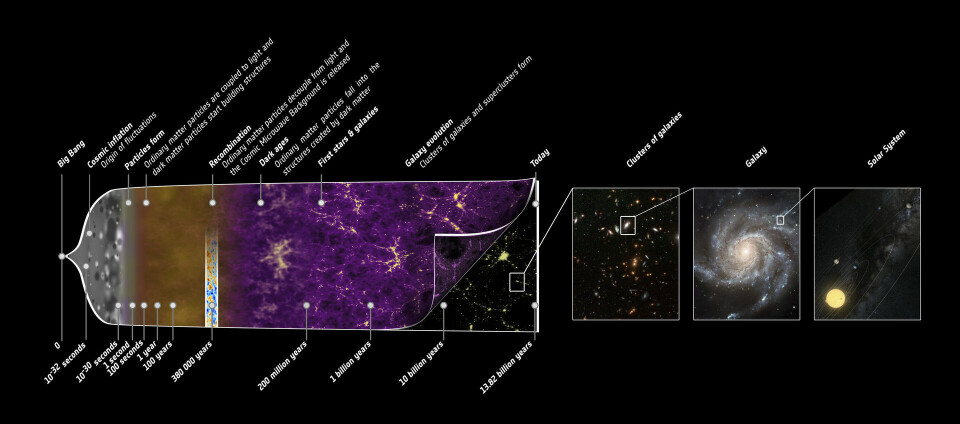
Watts explains that the biggest problem with measuring cosmic infrared background radiation is that the radiation is quite weak. At the same time, we live within our own solar system, inside the Milky Way, which is also filled with this cold, glowing dust.
Following galaxies through cosmic time
The best way to separate the Milky Way and dust in our solar system from the rest of the universe is to use many different datasets from various telescopes and combine them into a single model, Watts believes.
He plans to use historical data from various satellites, including the JAXA AKARI satellite, NASA’s IRA satellite, and the upcoming SPHEREx, which will be operational next year.
“These datasets cover several decades of space observations and have so far only been analysed separately. Now, we will bring the data together into a unified framework. This has never been done before,” says Watts.
If the researchers succeed, they will be able to present three-dimensional maps of space for different epochs. This way, we can study the development of the universe over time.
“One of the things that interests me the most is being able to see how the brightness of galaxies changes over time. Researchers have done this before with highly sensitive instruments, but they’ve only looked at very small areas at a time. What we're going to do is create maps of practically the entire sky and then divide it into different epochs. This way, we can follow galaxies as they form throughout cosmic time,” says Watts.
———
Read the Norwegian version of this article on forskning.no
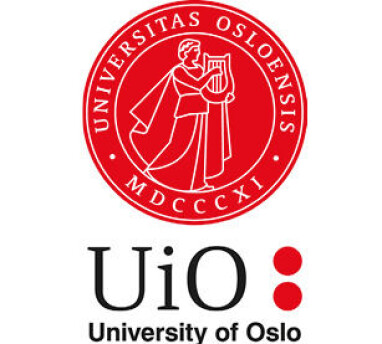
This content is paid for and presented by the University of Oslo
This content is created by the University of Oslo's communication staff, who use this platform to communicate science and share results from research with the public. The University of Oslo is one of more than 80 owners of ScienceNorway.no. Read more here.
More content from the University of Oslo:
-
Neuroscientist warns against the uncritical use of opioids
-
How Norway could succeed when a new crisis strikes
-
Melting glaciers are reducing freshwater supplies
-
Why are many early embryos lost?
-
Do you believe Antarctica is a pristine paradise? Think again
-
Do firefighters get prostate cancer because of their work?